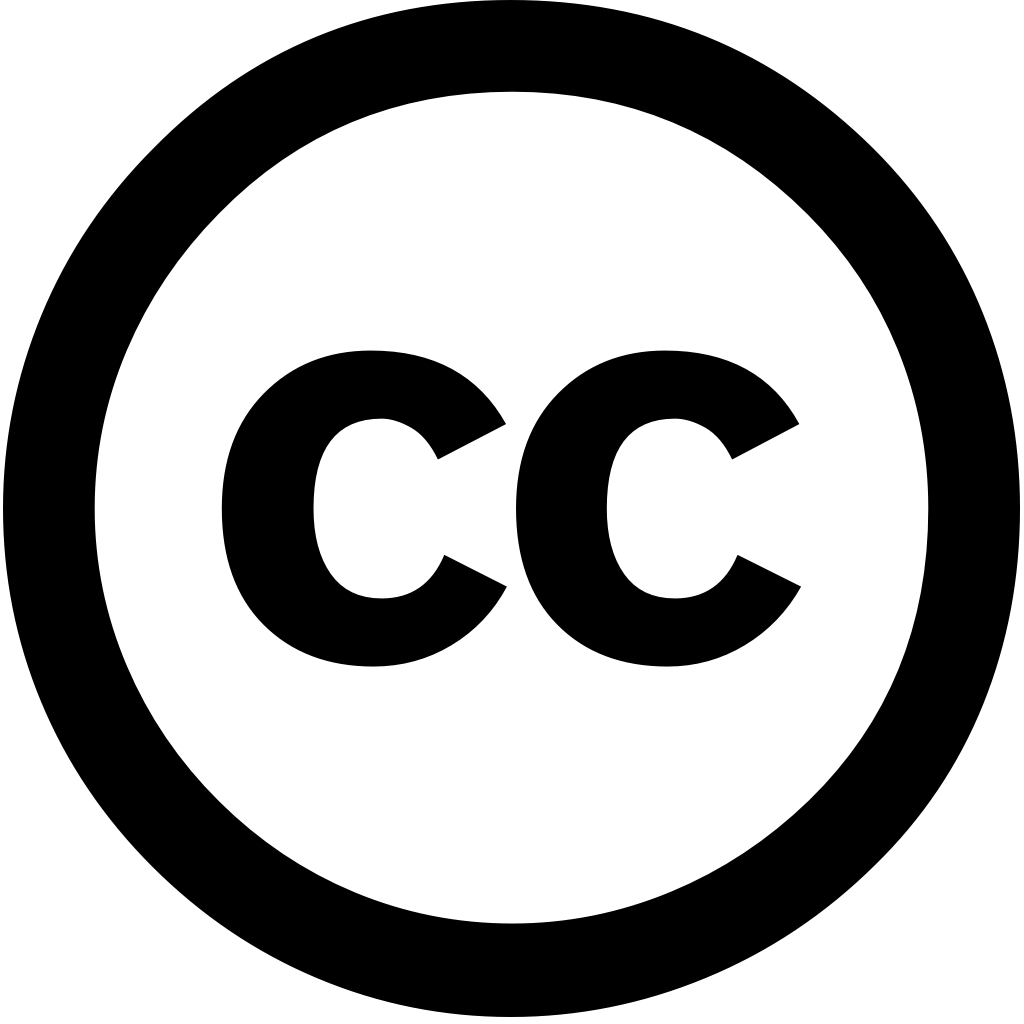
CCS Chemistry, Journal Year: 2021, Volume and Issue: 4(5), P. 1510 - 1518
Published: May 13, 2021
Open AccessCCS ChemistryCOMMUNICATION1 May 2022Nickel-Catalyzed Reductive Asymmetric Aryl-Acylation and Aryl-Carbamoylation of Unactivated Alkenes Youxiang Jin, Pei Fan Chuan Wang Jin Hefei National Laboratory for Physical Science at the Microscale Department Chemistry, University Technology China, Hefei, Anhui 230026 , School Chemical Materials Engineering, Huainan Normal University, Huainan, 232038 *Corresponding author: E-mail Address: [email protected] Center Excellence in Molecular Synthesis CAS, https://doi.org/10.31635/ccschem.021.202101040 SectionsSupplemental MaterialAboutAbstractPDF ToolsAdd to favoritesDownload CitationsTrack Citations ShareFacebookTwitterLinked InEmail Herein we report a nickel-catalyzed asymmetric two-component reductive aryl-acylation aryl-carbamoylation aryl-iodide-tethered unactivated alkenes, which utilize ortho-pyridinyl esters isocyanates as electrophilic acyl sources, respectively. Under catalysis nickel–pyrox complex with zinc powder reductant, variety chiral indanes, indolines, dihydrobenzofurans bearing quaternary stereogenic center were prepared moderate high efficiency good excellent enantioselectivities. The utility this method is demonstrated by various simple derivatizations attached carbonyl group, particularly sequential benzylic oxidation pinacol coupling, provide concise entry benzene-fused bicyclic bridged ring framework containing three challenging tetrasubstituted stereocenters stereocontrol. Download figure PowerPoint Introduction In last decade, transition metal-catalyzed enantioselective dicarbofunctionalizations tethered alkenes have evolved into reliable construct scaffold cyclic compounds.1–6 majority these reactions rely on facially selective Heck-type arylmetallation pendant olefinic unit enantiodetermining step,7 followed trapping generated σ-alkyl metal species either nucleophile or an electrophile. Based strategy, aryl-alkylation,8–10 diarylation,10–13 aryl-benzylation,14 aryl-alkenylation,15,16 aryl-alkynylation13,17,18 incorporated been developed under palladium, copper, nickel catalysis. As important subset dicarbofunctionalization reactions, olefin allows synthesis structurally compounds directly starting from considerable progress has accomplished domain.19–34 However, variants are still rare.31–34 2012, Dong et al.31 reported one-component version employing strained benzocyclobutanones precursors, was efficiently catalyzed rhodium (Figure 1a, equation 1). recent years, Correia32 Guan33 palladium-catalyzed carbonylative Heck using carbon monoxide combination aryl boronic acids anilines terminating agents, providing series highly enantioenriched carbonyl-containing oxindoles 2). Very recently, Ye co-workers34 described aryl-carbamoylation, successfully converted carbamoyl-fluoride-tethered wide range γ-lactams manner 3). Figure 1 | alkenes. aforementioned general approaches redox-neutral reaction pathway plagued one more following disadvantages: (1) requirement preformation organometallics; (2) cost noble (rhodium palladium) catalyst; (3) use toxic odorless gas, arguably undesirable laboratory scale synthesis; (4) basic conditions leading incompatibility base-sensitive functionalities. To address issues mentioned above, turned our attention establish strategy35–42 that could avoid pregenerated organometallics earth-abundant catalyst base-free conditions. We envisioned target would be achieved via appropriate source coupling partner aryl-halide-tethered stoichiometric reductant. This reductant offer new prepare benzene-annulated synthetically useful group fashion 1b). Results Discussion Optimization First, focused development aryl-acylation. realize reaction, initially screened acylating agents including ester 2a,43,44 acid chloride 2a- 1,45–50 fluoride 2,51 anhydride 3,52–54 thioester 4,55,56 activated amide 5,57 proven successful different cross-electrophile reactions43–57 (Table These alkene 1a performed DMA 40 °C NiBr2·glyme precatalyst, Pyrox L1 ligand, Zn best outcome terms both induction case 2a wherein desired product 3aa obtained 25% yield 95% ee. Table Preliminary Screening Electrophilic Acylating Agentsa–c aUnless otherwise specified, 0.2 mmol 2.0 equiv 2a–2a- 5, 10 mol % NiBr2·glyme, 15 0.5 mL 12 h. bYields isolated through column chromatography. cEnantiomeric excesses determined High-Performance Liquid Chromatography (HPLC) analysis stationary phase. Encouraged initial results, continued optimize varying parameters improve efficiency, first conducted higher temperature. Indeed, elevated 31% while enantioselectivity remained (Entry Subsequently, ligands examined studied (Entries 2–10). general, bis(oxazoline) (BiOX) L2– 8 able promote yields enantiomeric ranging 89% 2–8). contrast, bis(oxazoline)-pyridine (PyBOX) L9 phosphine–oxazoline (PHOX) L10 ligand failed deliver compound 9 10). Next, several Ni(II) salts bis(cyclooctadiene)nickel(0) (Ni(COD)2) surveyed 11–14), highest NiBr2·diglyme 14). case, also increased 38%. Although Ni(COD)2 gave yield, excess diminished 92% 13). At juncture, decided carry out 2 propensity undergo dimerization after cyclization. Gratifyingly, improved 61% based o-pyridinyl 15). A brief solvent screening undertaken, no better result provided 16–18). Performing 80 resulted but declined level 19). Replacing Mn reducing agent led inferior 20). Moreover, bromo analog precursor 3aa, albeit much lower 21). Reaction Conditionsa Entry Ligand Ni-precatalyst Solvent Yield (%)b ee (%)c 31 95 L2 27 55 3 L3 29 63 4 L4 24 5 L5 trace n.d.d 6 L6 11 89 7 L7 26 72 L8 35 0 – NiBr2 NiCl2 30 13 50 92 14 38 96 15e NiBr2· diglyme 61 16e DMF 41 17e NMP 60 94 18e THF 19 19e,f 64 20e,g 16 88 21h 32 2a, Ni-precatalyst, L cDetermined HPLC dNot determined. eThe preformed 1a. fThe °C. gMn used instead Zn. hThe analogue (2 equiv) Substrate scope After establishing optimum conditions, started investigate substrate Ni-catalyzed array benzoates n electron-donating electron-withdrawing substitution positions reacted standard delight, all proceeded smoothly, furnishing products 3aa– Of note organometallics- ( 3ah– am) nitrile 3an) moieties well tolerated, not previous arylboronic conditions.32,33 Furthermore, naphthoate posed problem, 3ao excess. 2-furonate 2p, -thiophenecarboxylate 2q, indole-2-carboxylate 2r proved pertinent enabling incorporation heterocycles backbone 3ap– ar) induction. Unfortunately, formed when alkanoates utilized precursors. Evaluation Scope Aryl-Acylationa–c 1, NiBr2·diglyme, dThe 4-mmol 2a. varied structure No significant impact observed increasing bulkiness geminal 3bd, 3bm, 3cd). Either groups introduced phenyl iodides, yielding 3fd, 3fm, 3gd, 3gm enantiocontrol. applicable construction indoline 3hd– jd) decreasing ascending N-substitution noticed. addition, simply scaled up mmol, affording 60% 94% introduce carbamoyl moiety across unit. chlorides unsuccessful. however, isocyanates58–60 competitive carbamoylating catalytic system slightly modified explored, results summarized 4. accommodated, benzamides 5aa– ak excesses. Notably, keto intact during reaction. aliphatic precursors products. On other hand, broad allowed efficient 5ba– oa) enantioselectivities most cases. Aryl-Carbamoylationa–c 1.5 4, L1, 1.0 NEt3 without NEt3. Derivatization cross-coupling demonstrate method, aryl-carbonylation subjected Wittig olefination, delivering 71% meta-Chloroperoxybenzoic (mCPBA)-mediated Baeyer–Villiger smoothly corresponding phenolic 64% yield. hydrolysis afforded carboxylic 88% present core GPR40 agonists61 ruthenium-catalyzed indane furnished set indanones yields.62 Taking advantage two 9, transformed them diverse rings centers diastereocontrol samarium-mediated intramolecular pinacol-coupling 2b).63 Besides, reduction 5aa LiAlH4 amine 75% 2c). one-pot aza-annulation styrene DDQ-mediated enabled installation quinolone onto 12) 2d).64 Derivatizations (a) mCPBA (10 equiv), para-toluenesulfonic monohydrate (pTsOH•H2O) (1 dichloroethane (DCE), °C, (b) LiOH (5 MeOH/H2O = 10:1, 45 (c) RuCl3 (0.16 %), iodide (PhI) oxone MeCN/H2O (1:1), room temperature, (d) Samarium (Sm) (1.2 trimethylsilyl THF, 67 20 Proposed mechanism basis reports,12,14,65 proposed plausible Initially, Ni(0) condition, underwent oxidative addition 1. resultant I arylnickelation unit, accomplish stereocenter. Zn-mediated intermediate II delivered Ni(I) III, addition. Facile elimination Ni(III) IV 3. Finally, o-pyridinylate V reduced Zn, regenerate next cycle. III migratory insertion C–N double bond install moiety. Reduction VI protonation completion yielded 5. mechanism. Conclusion conclusion, carbonylating agent, Ni/Pyrox key step. By circumventing organometallics, strong bases, functionality tolerance. further Particularly, stereocontrol oxidation/pinacol-coupling sequence. Supporting Information available includes experimental procedures, characterization data, 1H NMR, 13C 19F chromatograms (PDF). Conflict Interest authors declare competing interests. Acknowledgments work supported start-up funds Young Scholar 1000 Talents Plan, Natural Foundation China (21772183 22071230), Fundamental Research Funds Central Universities (WK2060190086), Project Funded Postdoctoral (2020M682006), China. References Giri R.; Shekhar K. C.Strategies Toward Dicarbofunctionalization Olefins Combined Carbometalation Cross-Coupling.J. Org. Chem.2018, 83, 1780–1788. Google 2. Derosa J.; Apolinar O.; Kang T.; Tran V. Engle M.Recent Developments Nickel-Catalyzed Intermolecular Alkenes.Chem. Sci.2020, 11, 4287–4296. Tu H.-Y.; Zhu S.; Qing F.-L.; Chu L.Recent Advances Three-Component Difunctionalization Alkenes.Synthesis2020, 52, 1346–1356. Qi X.; Diao T.Nickel-Catalyzed Alkenes.ACS Catal.2020, 10, 8542–8556. Luo Y.-C.; Xu C.; Zhang X.Nickel-Catalyzed Alkenes.Chin. J. Chem.2020, 38, 1371–1394. 6. Ping Y.; Kong W.Ni-Catalyzed 979–992. 7. Xie J.-Q.; Liang R.-X.; Jia Y.-X.Recent Catalytic Enantioselective Reactions Reductive‐Heck Reactions.Chin. Chem.2021, 39, 710–728. 8. Cong H.; Fu G. C.Catalytic Cyclization/Cross-Coupling Alkyl Electrophiles.J. Am. Chem. Soc.2014, 136, 3788–3791. 9. Y; C.Nickel-Catalyzed Arylalkylation Alkenes.Angew. Int. Ed.2019, 58, 6722–6726. 10. Z.-M.; B.; Wu L.; Qian Zhou Liu J.Enantioselective Palladium-Catalyzed Tandem Heck/Suzuki Coupling Reaction.Angew. 14653–14659. 11. You W.; Brown M. K.Catalytic Diarylation Alkenes.J. Soc.2015, 137, 14578–14581. 12. K.; Ding Z.; Activated Domino Cyclization/Cross-Coupling.J. Soc.2018, 140, 12364–12368. 13. J.-W.; Gao Z.-Y.; Y.-X.Stereoselective 1,2-Dicarbofunctionalization Trisubstituted Heck/Sonogashira Sequence.CCS 2, 2340–2349. 14. Yang Arylbenzylation Alkenes.Org. Lett.2020, 22, 2724–2729. 15. Tian Z.-X.; Qiao J.-B.; G.-L.; Pang Ma W.-Y.; Zhao Z.-Z.; Duan Du Y.-F.; Su P.; X.-Y.; Shu X.-Z.Highly Cross-Electrophile Aryl-Alkenylation Soc.2019, 141, 7637–7643. 16. Chen Li W.Nickel-Catalyzed Aryl Fluoroalkenylation Catal.2019, 9127–9133. 17. Bai Ge Lu Y.Pd/Cu‐Catalyzed Sequential Coupling: Oxindoles Containing Trifluoromethylated Quaternary Stereogenic Centers.Angew. Ed.2020, 59, 2764–2768. 18. Ji D.; Sequence.Angew. 2769–2775. 19. Dreis A. M.; Douglas C. J.Catalytic Carbon–Carbon σ Bond Activation: An Intramolecular Carbo-Acylation Acylquinolines.J. Soc.2009, 131, 412–413. 20. G.Rhodium-Catalyzed Regioselective Carboacylation Olefins: C–C Activation Approach Accessing Fused‐Ring Systems.Angew. Ed.2012, 51, 7567–7571. 21. Lutz Rathbun Stevenson S. Powell B. Boman T. Baxter E.; Zona Johnson B.Rate-Limiting Step Rh-Catalyzed Alkenes: Migratory Insertion.J. Soc.2012, 134, 715–722. 22. Ouyang X.-H.; Song R.-J.; J.-H.Iron-Catalyzed Oxidative 1,2-Carboacylation Alcohols: Route 3-(2-Oxoethyl)indolin-2-ones.Eur. Chem.2014, 2014, 3395–3401. 23. Wentzel Reddy Hyster J.Chemoselectivity C–H Controlling Hydroarylation Ed.2009, 48, 6121–6123. 24. Walker A.; Vickerman Humke N.; Stanley L. M.Ni-Catalyzed Alkene Amide Activation.J. Soc.2017, 139, 10228–10231. 25. Zheng Y.-L.; Newman G.Nickel-Catalyzed Heck-Type Using Methyl Esters Cross-Coupling Electrophiles.Angew. 18159–18164. 26. Arylacylation Carbonyl-Containing Oxindoles.Org. Lett.2019, 21, 7498–7503. 27. Oguma Miura Satoh Nomura M.Rhodium-Catalyzed Sodium Tetraphenylborate Acid Anhydrides Presence Absence Norbornene.J. Organomet. Chem.2002, 648, 297–301. 28. Kadam Metz Initiated Activation.ACS 5651–5656. 29. Fielding Grigg Urch J.Novel Carbamoyl Chlorides Palladium Catalysed Cyclisation–Anion Capture.Chem. Commun.2000, 36, 2239–2240. 30. Anwar U.; Sridharan V.; J.Palladium Catalyzed Capture Processes. Part 8: Situ Preformed Organostannanes. Carbamyl Other Starter Species.J. Chem.2006, 691, 1476–1487. 31. Ko H. Savage N. G.Highly Efficient Syntheses Chiral Poly-Fused Rings.J. 20005–20008. 32. Carmona R. Köster O. Correia D.Chiral N,N Ligands Enabling Palladium‐Catalyzed Heck–Matsuda Carbonylation CO Insertions.Angew. Ed.2018, 57, 12067–12070. 33. Kou Ren Z.-H.; Guan Z.-H.Palladium‐Catalyzed Monodentate Phosphoramidite Ligand: (+)‐Physostigmine, (+)‐Physovenine, (+)‐Folicanthine.Angew. 12199–12205. 34. F.-P.; R.-H.; S.-L.; Luan Y.-X.; M.Carbamoyl Fluoride-Enabled Ni-Catalyzed Carbocarbamoylation Soc.2020, 142, 19844–19849. 35. Everson D. Weix J.Cross-Electrophile Principles Reactivity Selectivity.J. 79, 4793–4798. 36. Moragas Correa Martin R.Metal-Catalyzed Organic Halides Carbonyl-Type Compounds.Chem. Eur. J.2014, 20, 8242–8258. 37. Gu Xue Gong H.Nickel-Catalyzed Electrophiles: Concept Mechanistic Considerations.Org. Front.2015, 1411–1421. 38. J.Methods Mechanisms Csp2 Electrophiles.Acc. Res.2015, 1767–1775. 39. Dai Couplings.Top. Curr. Chem.2016, 374, 43. 40. Richmond Moran J.Recent Nickel Catalysis Enabled Stoichiometric Metallic Reducing Agents.Synthesis2018, 50, 499–513. 41. Poremba Dibrell Reisman E.Nickel-Catalyzed Reactions.ACS 8237–8246. 42. Reactions.Synlett2020, 31, 1843–1850. Onaka Matsuoka Mukaiyama T.A Convenient Method Direct Preparation Ketones 2-(6-(2-Methoxyehtyl)pyridyl)carboxylates Iodies Use Zinc Dust Amount OF Dichloride.Chem. Lett.1981, 531–534. 44. Hoerrner Watson J.Nickel‐Catalyzed Dialkyl N‐Alkyl Pyridinium Salts Carboxylic Acids.Angew. 13484–13389. 45. Wotal J.Synthesis Functionalized Derivatives Halides.Org. Lett.2012, 14, 1476–1479. 46. F.; Q.; H.Ketone Formation Mild Chlorides.Org. 3044–3047. 47. Cherney Kadunce E.Catalytic Acyl Cross-Coupling: Enantioenriched Acyclic α,α-Disubstituted Ketones.J. Soc.2013, 135, 7442–744. 48. Guo L.Intermolecular Selective Radical Relay.Nat. Commun.2018, 3488. 49. Ring Opening Cycloketone Oxime Aroyl Chlorides.ACS Catal.2018, 8, 11324–11329. 50. C.Ni-Catalyzed 1,2-Iminoacylation Strategy.Org. Front.2018, 3476–3482. 51. Pan F.-F.; C.-L.; X.-Z.Enones Fluorides Vinyl Triflates Catalysis.Org. 3701–3705. 52. Yin Lin H.Mild Ketone Anhydrides.Chem. Commun.2012, 7034–7036. 53. H.Ni-Catalyzed Acids Tertiary Glycosyl Halides.J. 17645–17651. 54. Alkylacylation Electron-Deficient 8829–8835. 55. Ai Yahata Kishi Y.Zirconium/Nickel-Mediated One-Pot Synthesis.Angew. Ed.2017, 56, 10791–10795. 56. Cary Beyer P. Gellman J.Ketones Decarboxylative, Non-Symmetric Esters.Angew. 12081–12085. 57. Ni Mei Han Y.Ni-Catalyzed Amides Iodide Electrophiles Activation.Org. Lett.2017, 19, 2536–2539. 58. Hsieh J.-C.; Cheng Isocyanates 1,3-Iodoesters Halobenzenes: Novel Imide Derivatives.Chem. Commun.2005, 41, 4554–4556. 59. R.Ni-Catalyzed Amidation C–O Cleavage.J. 7253–7256. 60. Serrano R.Nickel‐Catalyzed Bromides.Angew. Ed.2016, 55, 11207–11211. 61. Dransfield Wong Kohn Yu Vimolratana A.-R.; Jiao (J.); Swaminath G.; Zhuang Connors Medina Houze B.Improving Pharmacokinetics GPR40/FFA1 Full Agonists.ACS Med. Lett.2014, 384–389. 62. Yusubov Nemykin Zhdankin V.Transition Metal-mediated Oxidations Utilizing Monomeric Iodosyland Iodylarene Species.Tetrahedron2010, 66, 5745–5752. 63. Ogawa Takeuchi Hirao T.Diastereoselective Pinacol Rare Earth Metals Chlorosilanes.Tetrahedron Lett.1999, 40, 7113–7114. 64. Huang Y.-H.; S.-R.; D.-P.; P.-Q.Intermolecular Dehydrative [4 + 2] Aza-Annulation N-Arylamides Divergent Entrance Aza-Heterocycles.Org. 1681–1685. 65. 6989–6994. Previous articleNext article FiguresReferencesRelatedDetails Issue AssignmentVolume 4Issue 5Page: 1510-1518Supporting Copyright & Permissions© 2021 Chinese SocietyKeywordsnickelreductive cross-couplingacylationcarbamoylationdicarbofunctionalizationAcknowledgmentsThis Downloaded 1,942 times PDF DownloadLoading ...
Language: Английский