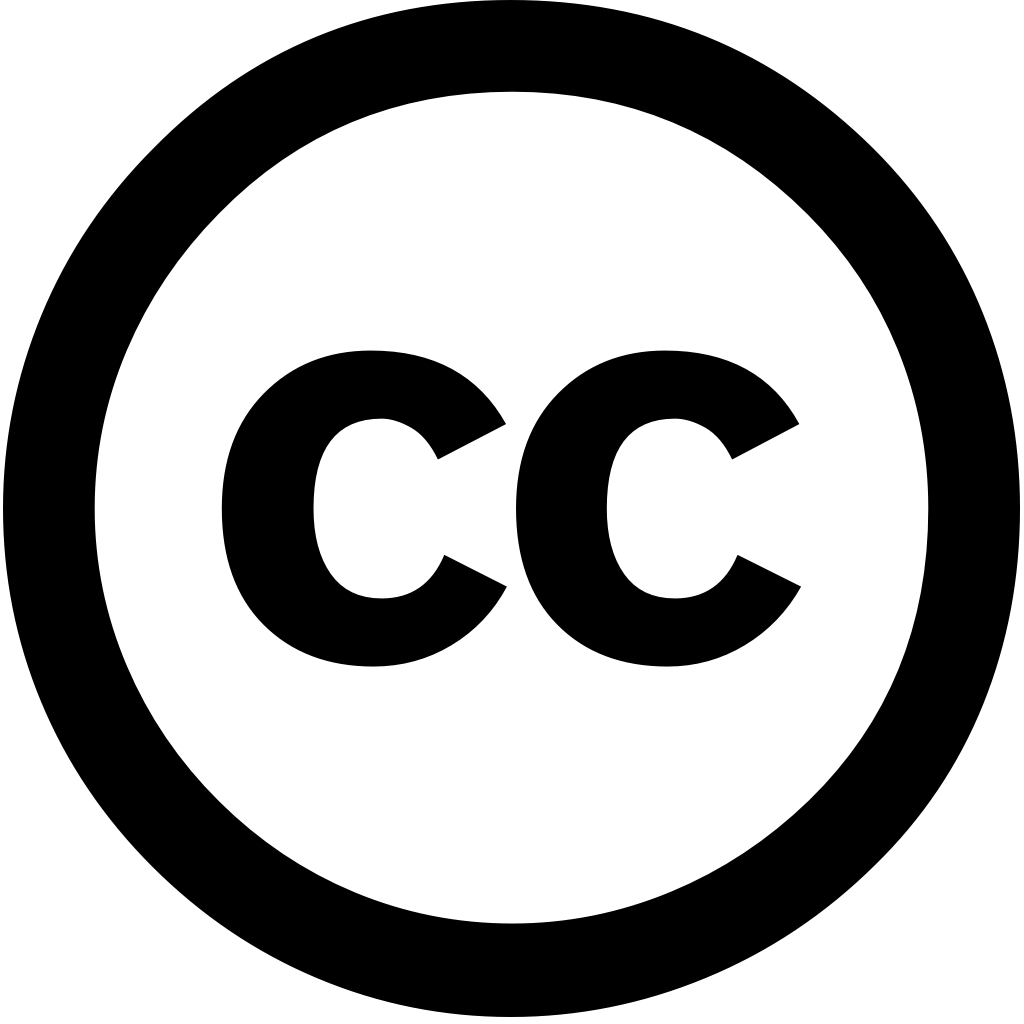
CCS Chemistry, Journal Year: 2020, Volume and Issue: 3(2), P. 1529 - 1552
Published: July 17, 2020
Open AccessCCS ChemistryMINI REVIEW1 Feb 2021Rhodaelectro-Catalyzed C–H and C–C Activation Youai Qiu†, Cuiju Zhu†, Maximilian Stangier, Julia Struwe Lutz Ackermann Qiu† Institut für Organische und Biomolekulare Chemie, Georg-August-Universität Göttingen, Göttingen 37077 , Zhu† Stangier *Corresponding author: E-mail Address: [email protected] https://doi.org/10.31635/ccschem.020.202000365 SectionsAboutAbstractPDF ToolsAdd to favoritesDownload CitationsTrack Citations ShareFacebookTwitterLinked InEmail Rhodium(III) catalysis has set the stage for a plethora of oxidative functionalizations over last decade, which have predominantly employed stoichiometric amounts toxic expensive metal oxidants, such as silver(I) salts. In meantime, electrosynthesis emerged an increasingly viable alternative oxidants. Recently, significant momentum been achieved with merger electrocatalysis organometallic activation. However, user-friendly robust rhodaelectro-catalysis until very recently proven elusive activations. This minireview highlights current knowledge recent advances electrooxidation in rhodium-catalyzed or activations, topical focus on contributions from group through July 2020. Download figure PowerPoint Introduction Organometallic activation one most efficient tools molecular synthesis.1–10 Particularly, rhodium(III) received notable attention development functionalizations.11–16 Despite considerable advances, rhodium(III)-catalyzed activations heavily rely and/or copper(II) salts sacrificial oxidants.17–21 identified strategy decade.22–36 While general reviews reports metallaelectro-catalysis appeared,37–59 state-of-the-art rhodaelectro-catalyzed transformations is not yet available,60,61 despite its unique potential syntheses, pharmaceutical industries, material sciences.62–65 Herein, we discuss developments specific interests mechanistic aspects. Thus, specifically summarize our findings provide number useful structures and, more importantly, reveal new synthetic disconnections. Overall, C−H/C–C syntheses levels resource economy.66 Rhodaelectro-Catalyzed C−H Alkenylation 2018, key breakthrough was established by (Göttingen, Germany) (Scheme 1).67 Hence, cross-dehydrogenative C–H/C–H alkenylation weakly O-coordinating68 benzoic acids 1 alkenes 2, serving proof concept first rhodium electrocatalyzed The optimized reaction conditions were characterized using Potassium acetate (KOAc) additive mixture t-AmOH H2O effective solvent system, delivering desired products 3 undivided cell setup. Initially, various substituents ortho-, meta-, para-positions probe robustness transformation, proceeding excellent positional, diastereo-, chemoselectivities. Notably, variety valuable electrophilic functional groups, including sensitive esters ketones, fully tolerated this electrooxidative alkenylations. Likewise, variously substituted acrylates 2 proved be amenable, oxidation-sensitive aliphatic hydroxy group. Furthermore, procedure applicable amides indoles. Specifically, endogenous steroid pregnenolone 2k could efficiently converted 3k without racemization stereogenic centers. It worth noting that electrochemical vinyl initially realized study, well.69,70 Scheme | Rhodaelectro-catalyzed alkenylation. Competitive experiments showed clear preference favor electron-rich 1. experiment conducted analysis initial rates electron-deficient 1c 1d independent reactions 2a). observation good agreement base-assisted intramolecular electrophilic-type substitution (BIES)71–78 manifold. deuteration studies CD3OD cosolvent suggested facile reversible event, while highlighting mechanism 2b). A minor kinetic isotopic effect illustrated rhodanation rate-determining step, providing additional support fast scission 2c). (a–c) Summary findings. On basis findings, catalytic cycle depicted proposed. carboxylate-assisted BIES delivers cyclometalated intermediate 4b. Next, migratory alkene insertion generates catalytically competent species 4d. Thereafter, β-hydride elimination reductive deliver 3. Finally, anodic oxidation reduced rhodium(I) 4e regenerates active 4a via single-electron transfer (SET) event. Plausible contrast α,β-unsaturated carbonyl compounds under rhodaelectro-catalysis, reported intriguing unactivated 6 coordinating benzamides 4).79 Here, dehydrogenative 7 obtained NaOPiv instead previously KOAc additive. shown proceed ample substrate scope, heterocycles group, chloro, bromo, nitrile. hydroxyl substituents. gram-scale highlighted utility 4 reaction. Alkynylation rhodaelectro-catalytic broadly gave access synthetically polycyclic aromatic hydrocarbons (PAHs)80–84 two-step sequential annulation 5a).85 C−B/C−H [2 + 2] cyclization boronic featuring versatile catalysis. scope remarkable tolerance, ester, cyano substituents, chemoselectivity conversion iodo-substituted significantly improved compared typical chemical AgOAc Cu(OAc)2 5b). 5 (a b) C–B/C–H annulation. Further transformation tetraphenyl naphthalenes into π-conjugation PAHs presence 20 mol % 2,3-Dichloro-5,6-dicyano-1,4-benzoquinone (DDQ) divided at room temperature 6).86,87 Thereby, late-stage diversification provided important derivatives. unambiguous structure cyclodehydrogenated product 11 confirmed X-ray diffraction analysis, revealing structurally nonplanar PAH. addition, photoabsorption cyclic voltammetry (CV) measurements reflected optoelectronic properties electrochemically generated 7).88,89 Late-stage DDQ-catalyzed cyclodehydrogenation. (a) Photoabsorption (b) CV 11e 11f. Importantly, also flow alkyne annulations aryl imidates 12 substrates 8).90 particularly noteworthy C−H/N−H amenable electroflow technology slightly modified IKA setup.91–98 represents tool upscaling control heat mass transfer. challenging isoquinolines, well azo-tetracycles, 8 Flow-rhodaelectro-catalyzed annulations.a [Cp*Rh(CH3CN)3](SbF6)2 (5.0 %) catalyst, 50 °C, 10 h, batch In-depth performed catalyst's modus operandi 8). synthesis two novel complexes 17a 17b 12a accomplished 9a). well-defined found 9b). formation well-characterized rhodium(III)-heptacycle 18 observed when treating complex 13a, whereas 13a underwent 9c). 14a electricity applied, thus oxidation-induced within unusual rhodium(III/IV/II) regime 9d).99,100 gain further insights role sodium salt. These indicated additive, NaOPiv, accelerated rhodacycle upon electrolysis. 9 (a–d) Synthesis rhodacyles 17a, 17b, 18, applications computational rationalized favorable Rh(III/IV/II) manifold barrier 15.2 kcal mol–1 oxidatively induced step (Figure 1). experimental Figure Gibbs free-energy profile (in mol–1) comparing direct B3LYP-D3(BJ)/6-311++G(d,p),SDD(Rh)+SMD(methanol)//B3LYP-D3(BJ)/6-31G(d,p),SDD(Rh) level theory. Nonparticipating hydrogen atoms omitted clarity. these studies, plausible proposed feature cyclometallated 17 10). SET rhodium(IV) C subsequently undergo C. 14 released, regenerated. Proposed flow-rhodaelectro-catalyzed Electrooxidative merged multiple domino 11).101 previous transformation,85 use easily accessible imidamides 19 enabled aza-PAHs. cascade tolerance. Having demonstrated versatility annulation, encouraged investigate 12). occurred rhodacycles 21 22 catalysts. order three subsequent events. An application dendrimer 23 assembly protected d-lactone 24 13). Key 13 functionalization Phosphorylation Xu group102 concurrently disclosed mechanistically related phosphorylation N-coordinating directing 14). utilized diphenylphosphines 26. To prove scalability, decagram scale successfully performed, illustrating future industrial applications. phosphorylation. interrogated detailed studies. 28 29 prepared. Both settings 15a). undergoes ligand exchange form oxidizable 29, followed generate 27 15b). 15 electrochemistry ideal platform proof-of-concept Chang group103 probed viability steps First, stable 33 35a prepared their investigated 16a). irreversible Epa = 0.331 V versus Fc/Fc+ tetrahydrofuran (THF), can oxidized silver salt putative high-valence according known AgI (E1/2 0.41 vs THF). Indeed, arylated methylated 37a 37b obtained, did take place even 80 °C oxidant-free conditions. confirm (Schemes 16b 16c). 16 Oxidatively complexes. C−C limited transformations. alkenylation, representing functionalization104 electrocatalysis.105 Within manifold, chelation-assisted outstanding chemo- position-based selectivities 17a). competition between revealed preferential reactivity position selectively furnishing densely decorated 1,2,3-substituted arenes, common strategies 17b). selectivity. mode action 18). Competition arenes olefins preferentially 18a). faster than 18b). isotopically labeled [D]1-tAmOD D2O lead unreacted starting product. are indicative slow 18c). sole byproduct cathodic proton reduction headspace gas chromatographic 18d). 42a 42b catalysts nature 18e). (a–e) Their 19. Initiated catalyst 4a, seven-membered 43 formed chelating nitrogen oxygen 38 catalyst. 46. furnished 40, regenerated 47. Conclusions years, powerful synthesis, employing sustainable terminal oxidant avoiding Since example O-coordinating described numerous elegant applying established. breakthroughs understanding overall among others analyses, CV, computation, prominently pathways. metallaelectro-catalyzed terms annulations. expedient Given reaction, exciting expected rapidly evolving research area, should address enantioselective metallaelectro-catalysis,106 photoelectrochemical transformations,107–112 organic materials syntheses. Conflict Interest authors declare no conflict interest. Acknowledgments Generous Deutsche Forschungsgemeinschaft (German Research Foundation) (Gottfried-Wilhelm-Leibniz award L.A.) China Scholarship Council (fellowship C.Z.) gratefully acknowledged. References Park Y.; Kim S.Transition Metal-Catalyzed Amination: Scope, Mechanism, Applications.Chem. Rev.2017, 117, 9247–9301. Google Scholar 2. Gandeepan P.; L.Transient Directing Groups Transformative Synergistic Metal Catalysis.Chem2018, 4, 199–222. Piou T.; Rovis T.Electronic Steric Tuning Prototypical Piano Stool Complex: Rh(III) Catalysis Functionalization.Acc. Chem. Res.2018, 51, 170–180. 4. Wei Hu Zhang M.; Su W.Metal-Catalyzed Decarboxylative Functionalization.Chem. 8864–8907. 5. He J.; Wasa Chan K. S. L.; Shao Q.; Yu J.-Q.Palladium-Catalyzed Transformations Alkyl Bonds.Chem. 8754–8786. 6. Wencel-Delord Glorius F.C–H Bond Enables Rapid Construction Late-Stage Diversification Functional Molecules.Nat. Chem.2013, 5, 369–375. 7. Arockiam P. B.; Bruneau C.; Dixneuf H.Ruthenium(II)-Catalyzed Rev.2012, 112, 5879–5918. 8. L.Carboxylate-Assisted Transition-Metal-Catalyzed Functionalizations: Mechanism Scope.Chem. Rev.2011, 111, 1315–1345. 9. Davies H. M. Manning J. R.Catalytic Functionalization Carbenoid Nitrenoid Insertion.Nature2008, 451, 417–424. 10. Bergman R. G.C–H Activation.Nature2007, 446, 391–393. 11. Shin K.; H.; S.Transition-Metal-Catalyzed C–N Forming Reactions Using Organic Azides Nitrogen Source: Journey Mild Versatile Amination.Acc. Res.2015, 48, 1040–1052. 12. Sperger Sanhueza I. A.; Kalvet I.; Schoenebeck F.Computational Studies Synthetically Relevant Homogeneous Involving Ni, Pd, Ir, Rh: Overview Commonly Employed DFT Methods Mechanistic Insights.Chem. Rev.2015, 115, 9532–9586. 13. Kuhl N.; Schroeder F.Formal SN-Type Rhodium(III)‐Catalyzed Activation.Adv. Synth. Catal.2014, 356, 1443–1460. 14. Song G.; Wang F.; Li X.C–C, C–O Formation Rhodium(III)-Catalyzed Oxidative Activation.Chem. Soc. 41, 3651–3678. 15. Colby D. Ellman A.Rhodium-Catalyzed Heteroatom-Directed Rev.2010, 110, 624–655. 16. Satoh Miura M.Oxidative Coupling Aromatic Substrates Alkynes Alkenes Under Rhodium Catalysis.Chem. Eur. J.2010, 16, 11212–11222. 17. Liu Yang Shi X.; Cheng You J.Rhodium-Catalyzed Benzoic Acids Terminal Alkynes: Efficient Access 3-Ylidenephthalides.Organometallics2016, 35, 1350–1353. 18. Kudo E.; Shibata Yamazaki Masutomi Miyauchi Fukui Sugiyama Uekusa Annulation Arenecarboxylic Acrylic Ambient Conditions Catalyzed Electron‐Deficient Complex.Chem. J.2016, 22, 14190–14194. Rajamalli H.Rhodium(III)‐Catalyzed [4+1] Vinylic Carboxylic Allenes: Method Towards Vinyl‐Substituted Phthalides 2‐Furanones.Chem. J.2015, 21, 9198–9203. 20. Xie Huang H.An Rh/O2 Catalytic System Activation/Annulation: Evidence Rh(I) Oxidation Molecular Oxygen.J. Am. Soc.2013, 135, 8850–8853. 21. Ueura M.An Waste-Free Regioselective Cleavage: Rh/Cu-Catalyzed Reaction Acrylates Air.Org. Lett.2007, 9, 1407–1409. 22. Zhu Oliveira Massignan L.Iron-Electrocatalyzed Arylations: Insights Oxidation-Induced Reductive Elimination Ferraelectrocatalysis.Chem. J.2019, 25, 16382–16389. 23. Xiong H.-C.Chemistry Electrochemically Generated N-Centered Radicals.Acc. Res.2019, 52, 3339–3350. 24. Parry Fu Lin S.Electrocatalytic Difunctionalization Olefins General Approach Vicinal Diamines.Synlett2018, 257–265. 25. Möhle S.; Zirbes Rodrigo Gieshoff Wiebe Waldvogel R.Modern ElectroChemical Aspects Value‐Added Products.Angew. Int. Ed.2018, 57, 6018–6041. Sauermann Meyer T. Qiu L.Electrocatalytic Activation.ACS Catal.2018, 8, 7086–7103. 27. Tang Lei A.Electrochemical Cross-Coupling Hydrogen Evolution: Green Sustainable Way Formation.Chem2018, 27–45. 28. Feng R.; Smith Moeller D.Anodic Cyclization Strategies Enable Optimization.Acc. Res.2017, 50, 2346–2352. 29. Yan Kawamata Baran S.Synthetic Electrochemical 2000: Verge Renaissance.Chem. 13230–13319. 30. Hou Z.-W.; Mao Z.-Y.; H.-C.Recent Progress (aza) Indoles Through Alkyne Reactions.Synlett2017, 28, 1867–1872. 31. Jiao K.-J.; Zhao C.-Q.; Fang Mei T.-S.Palladium CH Oxidation.Tetrahedron Lett.2017, 58, 797–802. 32. Horn E. Rosen B. Electrochemistry: Enabling Innately Method.ACS Cent. Sci.2016, 302–308. 33. Francke Little D.Redox Electrosynthesis: Basic Principles Recent Developments.Chem. Rev.2014, 43, 2492–2521. 34. Janza B.Renaissance Electrosynthetic Complex Molecules.Angew. Ed.2014, 53, 7122–7123. 35. Jutand A.Contribution Electrochemistry Rev.2008, 108, 2300–2347. 36. Yoshida J.-i.; Kataoka Horcajada Nagaki A.Modern Electroorganic Synthesis.Chem. 2265–2299. 37. L.Metalla-Electrocatalyzed Earth-Abundant 3d Metals Beyond.Acc. Res.2020, 84–104. 38. Scheremetjew Finger L. L.Electrophotocatalytic Undirected Trifluoromethylations (Het)Arenes.Chem. J.2020, 26, 3241–3246. 39. Shrestha Lee Dunn A. Sanford S.Palladium-Catalyzed Acetoxylation Oxidation.Org. Lett.2018, 20, 204–207. 40. Y.-J.; H.-C.Ruthenium-Catalyzed Dehydrogenative Annulation.ACS 3820–3824. 41. Tian Rogge L.Electrooxidative Ruthenium-Catalyzed C–H/O–H Weak O-Coordination.Angew. 5818–5822. 42. Sau Ang N. W. Allene Annulations Cobalt-Catalyzed 9140–9147. 43. L.Electrochemical C–H/N–H Water-Tolerant Cobalt Room Temperature.Angew. 2383–2387. 44. L.Electroremovable Traceless Hydrazides Electro-Oxidative Internal Alkynes.J. Soc.2018, 140, 7913–7921. 45. Ye K.-Y.; Pombar Sauer G. Keresztes S.Anodically Coupled Electrolysis Heterodi-functionalization Alkenes.J. 2438–2441. Gao Zeng A.Cobalt (II)-Catalyzed Amination Arenes Alkylamines.J. 4195–4199.
Language: Английский